Key takeaways:
~Carnitine is necessary for transporting long-chain fatty acids into mitochondria for energy production, which is particularly important in skeletal muscles and heart function.
~ Beyond energy production, carnitine plays roles in liver health, bone health, and immune function, and has anti-inflammatory properties.
~ The body can synthesize carnitine from lysine and methionine (requiring vitamin C, B6, and iron), or obtain it from diet, with meat and dairy being the richest sources.
~ Genetic variants in carnitine-related genes (SLC22A5, CPT1, CPT2) can cause disorders that affect energy production, causing issues from mild fatigue to severe dysfunction.
What Is Carnitine, and Why Is It Important?
Carnitine is a molecule made in the body that is important for normal mitochondrial function and energy production. Most importantly, it facilitates the transport of long-chain fatty acids into the mitochondria for use in ATP production. Carnitine also plays a role in metabolic regulation, detoxification, and cell membrane stabilization.[ref]
Let’s take a look at the many ways carnitine is used in the body and how your genetic variants may affect energy production.
Carnitine in Mitochondrial Energy Production
Mitochondria produce ATP, which is used for cellular energy, through a process called oxidative phosphorylation. This process can start with carbohydrates or fats.
- The body breaks down carbohydrates into glucose, which is then further broken down into acetyl-CoA.
- The body can also break down fatty acids of various lengths to finally form acetyl-CoA, which is used in the Krebs cycle to make ATP.
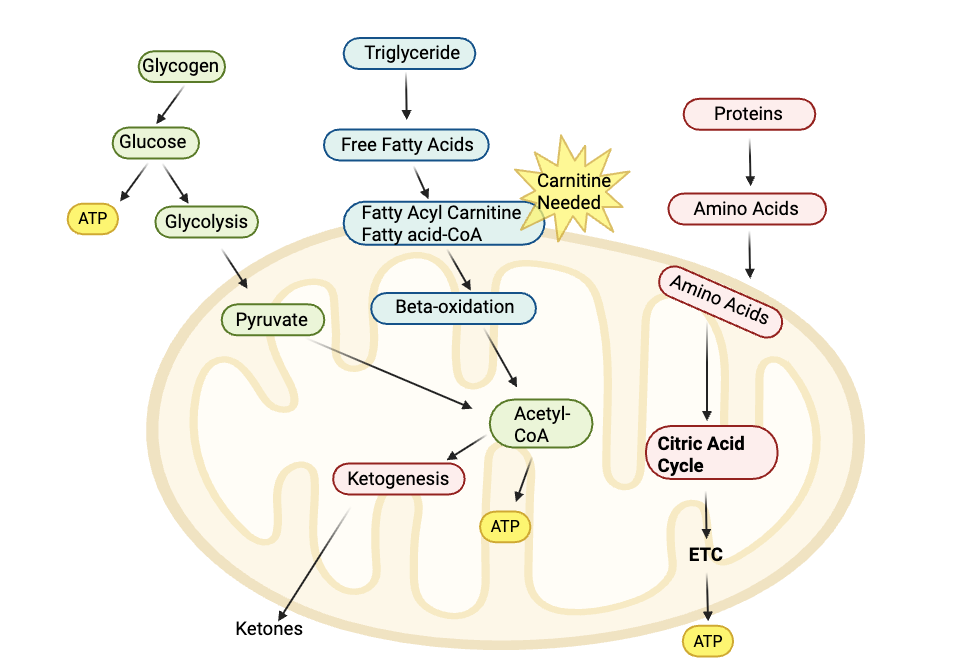
Carnitine plays an essential role in the production of energy from fat in the mitochondria. Without sufficient carnitine (along with specific enzymes), long-chain fatty acids can’t be transported into the mitochondria.
Let’s take a look at how this process takes place, including some of the genes/enzymes involved.
The Carnitine Shuttle: Moving long-chain fatty acids into and out of the mitochondria
The carnitine shuttle is a three-step transport system that enables long-chain fatty acids to cross the inner mitochondrial membrane for β-oxidation. Since the membrane is impermeable to these fatty acids, carnitine acts as a carrier via the following steps:[ref]
- Carnitine is bound to the fatty acyl group using CPT1 (carnitine palmitoyltransferase 1) to form acylcarnitines at the outer mitochondrial membrane.
- Acylcarnitines are shuttled across the inner mitochondrial membrane by CACT (carnitine-acylcarnitine translocase).
- Carnitine is removed from the acyl group by CPT2 (carnitine palmitoyltransferase 2), and the free acyl-CoA can then be used for ATP production.
- Carnitine is then shuttled back outside the mitochondria to the cytoplasm via CACT.
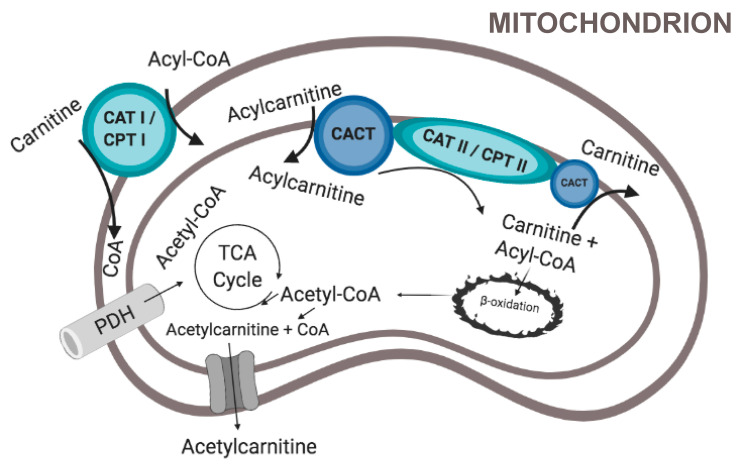
Again, this is the way that long-chain fatty acids are moved into the mitochondria, where β-oxidation breaks them into acetyl-CoA for ATP production.
Medium and short-chain fatty acids don’t need a transport system to move into the mitochondria.
Fatty acids and energy needs:
Fatty acids are used in the mitochondria to produce energy when glucose isn’t available. Skeletal muscles and the heart are both major energy consumers.
Skeletal muscles switch to fatty acid oxidation when they are in use, such as lifting weights, aerobics, sports, etc, but when at rest, they have a low rate of fatty acid oxidation. On the other hand, the heart uses fatty acid oxidation regularly, with 50-70% of energy coming from fatty acids.[ref]
Other roles of carnitine in the mitochondria:
In addition to transporting long-chain fatty acids into the cell, carnitine is also involved in moving certain metabolites out of the mitochondria. In addition, carnitine works to balance the levels of coenzyme A (CoA) in your mitochondria. Essentially, CoA needs to be at the right amount in the mitochondria.[ref]
Recent research shows that carnitine, as acetylcarnitine, helps to improve mitochondrial biosynthesis and overall function. In general, acetylcarnitine can also act as an antioxidant to reduce high levels of ROS in the mitochondria. However, abnormally high long-chain acetylcarnitines can aggravate mitochondrial damage. Having sufficient carnitine helps to keep the balance right and mitochondria functioning properly.[ref]
How is carnitine synthesized?
Carnitine can be synthesized in the body or obtained from food (mainly meat and dairy).
Carnitine synthesis involves the amino acids lysine and methionine. It is synthesized primarily in the liver, kidneys, and brain. The synthesis process is a multi-step process involving several enzymes that convert trimethyl-lysine to L-carnitine. The final step in L-carnitine synthesis occurs primarily in the liver, and liver dysfunction decreases L-carnitine levels.
Adequate iron, vitamin C, and vitamin B6 are also necessary. TMLH (N-trimethyllysine dioxygenase) is used in the first step of synthesis, along with iron and vitamin C. The second step involves 3-hydroxy-Nε-trimethyllysine aldolase enzyme (HTMLA gene) along with vitamin B6 as a cofactor.[ref]
Carnitine absorption and cellular transport: SLC22A5
Carnitine is transported into cells using the OCTN2 transporter or through passive transport. OCTN2 is encoded by the SLC22A5 gene. Rare mutations in the SLC22A5 gene can cause a genetic condition called primary carnitine deficiency, which is usually discovered in infancy because it affects brain function, muscle function, and blood glucose levels. [ref]
The highest sources of L-carnitine in the diet are meat and dairy products, with very little found in plant sources. People who are vegetarians mainly rely on their endogenous production of carnitine.[ref]
While the OCTN2 transporter is important in carnitine uptake, in some tissues, such as the lungs and intestines, carnitine can also be absorbed by passive diffusion.[ref][ref]
The expression of the OCTN2 transporter is regulated by estrogen receptors and PPARs. It is tightly controlled to prevent uncontrolled fatty acid oxidation in certain types of cancer cells.[ref]
Carnitine and Bone Health:
Carnitine is important in the way that macrophages function in the immune response and in bone health in aging. People with osteoporosis are likely to have lower carnitine levels in their macrophages in their bones. One study found that increasing carnitine levels helped to balance the macrophages in the bone marrow, resulting in a more balanced breakdown and rebuilding of bone. Supplemental carnitine may be helpful in osteoporosis.[ref]
Carnitine in NAFLD and liver health:
Non-alcoholic fatty liver disease (NAFLD) affects about one-third of the population in Western countries. It develops when there is excess fat in liver cells due to too much fatty acid uptake in the liver, excess synthesis of fat in the liver, and not enough fat exported out of the liver. Because carnitine is involved in the metabolism of long-chain fatty acids, a carnitine deficiency may contribute to the development of fatty liver.
There are several reasons that researchers believe low carnitine plays a role in developing NAFLD. People with genetic mutations related to low carnitine absorption often end up with liver problems that are similar to NAFLD, and those liver problems can be reversed with L-carnitine. Similarly, drugs that inhibit the carnitine shuttle cause fatty liver. A number of studies show that L-carnitine supplementation reduces liver markers in NAFLD.[ref]
Carnitine for Brain Health:
The brain uses a lot of energy, and enhancing mitochondrial function in the brain by increasing carnitine may be helpful in several specific situations.
Stress:
Animal studies show that psychosocial stress causes oxidative stress in the brain. Increased L-carnitine reversed the oxidative stress.[ref] Psychosocial stress would include things like stress from relationships, work, and finances.
Narcolepsy:
Low acylcarnitine levels are found in patients with narcolepsy, which is a sleep disorder that causes daytime drowsiness as well as a sudden loss of muscle tone (cataplexy). Narcolepsy is strongly associated with certain variants in immune function, but researchers also find that acylcarnitine levels are low and variants in the CPT1B gene add to susceptibility to narcolepsy.[ref]
Alzheimer’s:
A number of studies have looked at L-carnitine or acetyl-L-carnitine supplementation for Alzheimer’s or mild cognitive impairment. The studies have mixed results. A recent review found that the benefits seen in some studies are likely due to the positive effects on the liver and thus the gut-liver-brain axis.[ref]
Depression:
Animal studies show that acetyl-L-carnitine has antidepressant effects through epigenetic mechanisms. In patients with major depressive disorder, there is a decrease in acetyl-L-carnitine levels, on average. This was especially true in patients with treatment-resistant depression.[ref] Some clinical trials also show that acetyl-l-carnitine supplementation is more effective than placebo in improving many symptoms of depression.[ref]
Carnitine in the immune system:
Carnitine also plays a role in inflammation and the immune response. In general, it has anti-inflammatory properties by inhibiting the activity of NF-κB by provoking an increase in miR-373 (a microRNA that affects NF-κB levels).[ref]
In the intestines, acetyl-L-carnitine has been shown to improve gut inflammation. In IBD, acetyl-L-carnitine improves immune tolerance by decreasing the ratio of Th17 cells and macrophages.[ref]
Inborn errors of metabolism: Genetic mutations and carnitine
Genetic mutations in the genes involved in the carnitine shuttle can cause inborn errors of metabolism for long-chain fatty acids.
Inborn errors of metabolism are genetic problems with enzymes involved in metabolic functions. There are a bunch of other inborn errors of metabolism, including short-chain acyl-CoA dehydrogenase deficiency and medium-chain acyl-CoA dehydrogenase deficiency.[ref]
Let’s look at the inborn errors of metabolism involving carnitine:
CPT2 gene:
The CPT2 gene encodes the enzyme that moves fatty acids attached to carnitine into the inner membrane of the mitochondria.
The CPT2 gene has a polymorphism found in about 1% of the population. The change in the DNA causes the enzyme to be more thermolabile, which means that the enzyme loses its activity when exposed to moderate heat. For this specific variant, temperature increases, such as having a fever, exposure to a sauna, or during heatstroke, can cause the CPT2 enzyme not to function. During normal body temperature, the half-life of the enzyme is shorter than normal, leading to a decrease in fatty acid beta-oxidation and ATP generation. [ref][ref]
Rare mutations in CPT2 cause carnitine palmitoyltransferase II (CPT II) deficiency. This is an autosomal recessive disorder, meaning that two mutations are necessary to have the severe disorder. CPT2 deficiency presents with a range of symptoms, from a severe form with infant (or neonatal) problems, including heart, liver, or kidney abnormalities or failure. Less severe forms include muscle pain and weakness as well as hypoglycemia. The form that affects muscles – myopathic form – may not be diagnosed until childhood or even early adulthood. It causes muscle weakness and rhabdomyolysis after hard exercise, fever, or prolonged fasting.[ref]
CPT1 deficiency:
There are two genes that encode the CPT1 gene: CPT1A and CPT1B. The CPT1A form is mainly found in the liver, while the CPT1B form is predominantly found in the heart and skeletal muscles.
Mutations in CPT1 can cause carnitine palmitoyltransferase I (CPT I) deficiency, which is an autosomal recessive genetic condition (need two copies of the mutation). However, carriers of single copies of mutations rarely have noticeable negative effects.[ref]
Primary carnitine deficiency:
The SLC22A5 gene encodes the carnitine transporter known as OCTN2. While some carnitine can move passively into cells, for optimal cellular function or for times of physical stress, the OCTN2 transporter is essential.
Rare mutations in SLC22A5 can cause hypoglycemia and liver problems from infancy, and this is one of the genetic conditions that is usually screened for at birth. Less severe SLC22A5 mutations can cause skeletal muscle problems or cardiomyopathy. The treatment involves high doses of carnitine, which can then enter cells using another amino acid transporter.[ref]
Epigenetic connections:
Carnitine and acetylcarnitine also play a role in epigenetics. Carnitine inhibits histone deacetylase, which is important in how genes are turned on or off in the nucleus of the cells. Acetylcarnitine levels in cells can directly affect the acetylation of certain genes and thus regulate cellular function when fatty acid oxidation is high.[ref]
MicroRNAs are short strands of RNA that attach to mRNA and regulate how much of a protein is expressed. In this case, miRNAs can alter the gene expression of the CPT2 and CPT1 genes, which in turn affect mitochondrial function and fatty acid oxidation.[ref] Similarly, supplemental L-carnitine can affect inflammatory pathways through altering the expression of microRNAs that in turn decrease inflammatory cytokines.[ref]
Genotype report: Carnitine
Lifehacks:
Let’s dig into the research on supplemental carnitine and go through some of the benefits as well as possible drawbacks.
Sauna and hot bath caution: CPT2
The CPT2 variants that are thermolabile (break down with heat) increase the risk of serious heatstroke when exposed to high heat. This can be from being outdoors and in the heat too long on a hot day, and there are reports of problems from sauna or hot steam bath exposure. Problems with heatstroke are exacerbated by the CPT2 variant, but this is more common in older adults (e.g. over 70).[ref]
Supplemental L-carnitine:
L-carnitine and acetyl-L-carnitine are readily available as supplements and may be beneficial for someone with carnitine-related genetic variants. While both types of supplements can increase carnitine levels, acetyl-L-carnitine has a little better absorption and may cross the blood-brain barrier more effectively.[ref]
Carnitine is also available as an injectable or IV, available at health spas or clinics. Talk with your healthcare provider if you have questions on whether it is right for you.
Studies on supplementing with carnitine:
Clinical trials show that supplemental L-carnitine or acetyl-L-carnitine may be beneficial outside of the narrow role in genetic deficiencies.
Taking L-carnitine for athletic performance or muscle gains:
Studies on L-carnitine for increasing muscle mass have very mixed results, with most studies showing that it doesn’t give a big benefit for resistance training.[ref] The benefit of supplemental carnitine is that it can help to reduce soreness and repair muscle damage more quickly.[ref]
- In male vegetarians (low dietary carnitine), supplementing with 2g/day for 12 weeks increased skeletal muscle carnitine levels but didn’t increase muscle mass. Note that this was just supplementation alone and not combined with weight lifting or exercise.[ref]
- In a study involving both young athletes and untrained, middle-aged adults, carnitine supplementation increased carnitine levels in the muscles.[ref]
- For acute aerobic performance, oral supplementation with 1-3g/day of carnitine gives a slight edge, but it doesn’t affect more moderate intensity athletic performance.[ref]
Supplemental L-carnitine in old age:
Related Articles and Topics:
Pyruvate Dehydrogenase Deficiency – Mitochondrial Dysfunction and Lactate Production