Key takeaways:
~ Parkinson’s disease risk includes a combination of genetic factors and environmental exposures.
~ In Parkinson’s and in Lewy Body dementia, damage occurs to dopaminergic neurons in a specific region of the brain.
~ Several different genetic pathways can contribute to the development of the diseases.
~ This article discusses various genetic variants that increase the risk of Parkinson’s disease and how they interact with environmental and lifestyle factors.
Members will see their genotype report below, plus additional solutions in the Lifehacks section. Consider joining today.
Is Parkinson’s Disease Hereditary?
Parkinson’s disease (PD) is a neurological disorder caused by the degradation of dopamine-producing neurons in a part of the brain called the substantia nigra. PD affects up to 10 million people worldwide.
Parkinson’s disease is a multisystem neurodegenerative disease involving the central nervous system, autonomic nervous system, enteric nervous system, and immune system. It involves both motor and non-motor symptoms.[ref]
Symptoms of Parkinson’s include motor system symptoms, such as rigidity, problems with balance, stiffness and slowness in walking, slowness in moving limbs, changes in handwriting, resting tremors (usually in the hand), and constipation. Symptoms can vary between individuals.
PD can also involve non-motor symptoms such as sleep disorders, autonomic dysfunction, gastrointestinal problems, and emotional dysregulation. People with Parkinson’s may have hallucinations, memory loss, weight loss, and eventually dementia. Loss of the sense of smell is often an early symptom that may happen several years before any of the motor symptoms.[ref][ref][ref]
Parkinson’s disease is caused by a loss of dopaminergic neurons in the substantia nigra. Parkinsonism is a term that describes the rigidity and slowness of movements that occurs when over 60% of the presynaptic dopaminergic neurons no longer function. Brain imaging shows Parkinson’s disease by identifying the loss of the dopamine transporter proteins in the brain.[ref]
Within the substantia nigra, the aggregation of alpha-synuclein proteins, oxidative stress, and neuroinflammation are through to play a role in the degeneration of the dopaminergic neurons. Lewy bodies and Lewy neurites are protein clumps that accumulate in the brain and contain alpha-synuclein.[ref] Lewy body pathology has also been detected in the olfactory (nose) epithelium in a percentage of patients with Parkinson’s disease.[ref][ref]
What is the substantia nigra?
The substantia nigra is a darker region of the brain that runs from above the brainstem down to the spinal cord. It is darker than the rest of the brain because it contains melanin (pigment that colors skin and hair).[ref]
Mitochondrial dysfunction and an inability to clear out damaged mitochondria are thought to play a causal role in neuronal cell death in the substantia nigra. Alpha-synuclein misfolding is accelerated by cardiolipin, which is produced in the mitochondrial inner membrane. Misfolded alpha-synuclein then causes hyperactive, persistent mitochondrial respiration (which increases oxidative stress).[ref][ref]
I’ll go into some of the environmental risk factors for Parkinson’s below, and you’ll see that many of them could lead to mitochondrial dysfunction, oxidative stress, and neuronal inflammation.
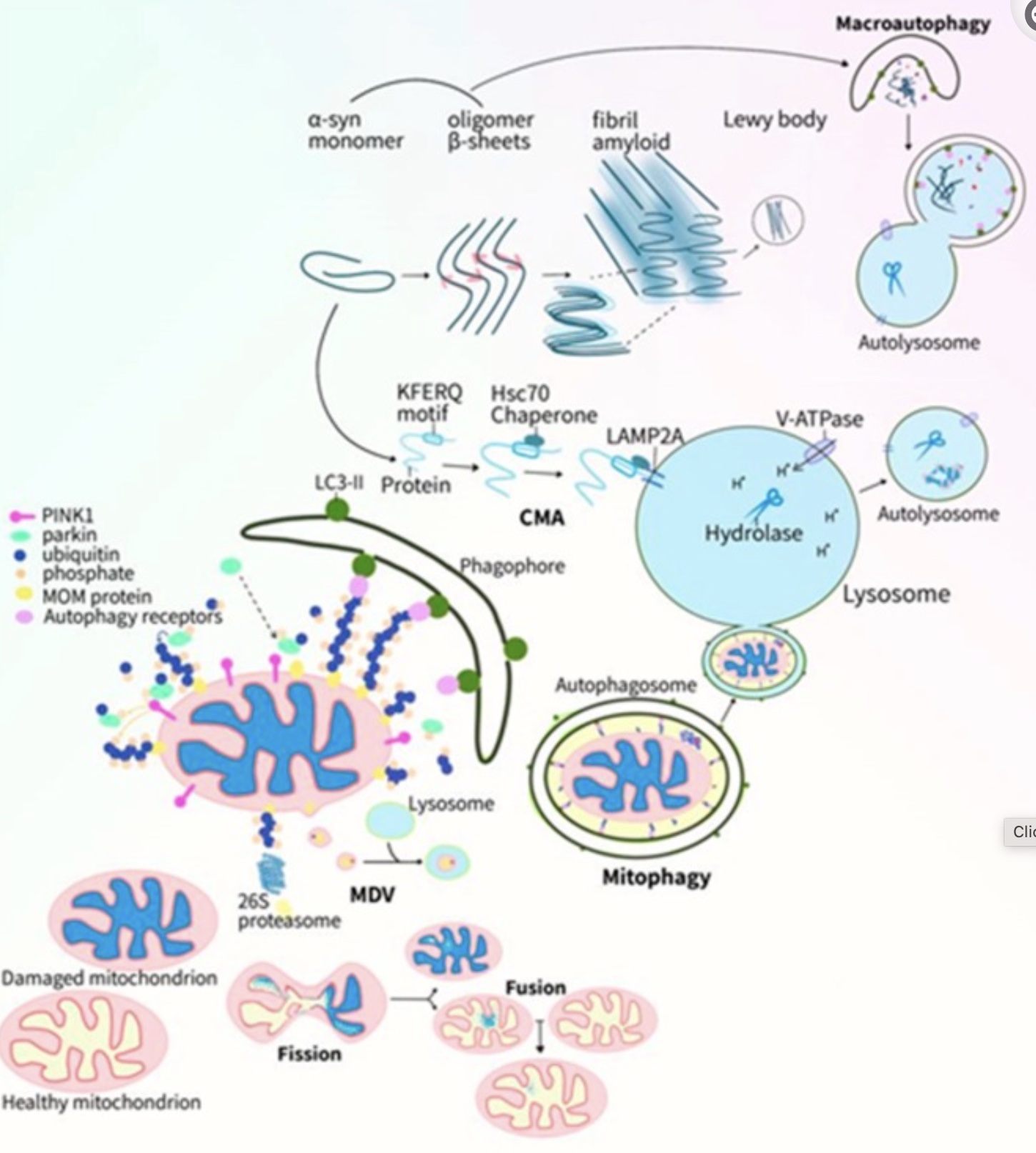
Parkinson’s disease or a Parkinsonian disorder?
The symptoms of parkinsonian disorders include disorders related to striatal dopaminergic deficiency, such as Lewy body dementia, and the term also applies to movement disorders such as secondary parkinsonism due to medications or adult-onset dystonic tremor. Neurologists can do a number of different tests to determine which disorder is causing the symptoms.[ref]
While the underlying causes of Parkinson’s disease (PD) are not yet completely understood, research shows it is caused by a combination of genetics and environmental factors for most people. (See the genotype report section for some of these genes)
There are two categories used for Parkinson’s:
- Early-onset Parkinson’s: Several rare genetic mutations, such as in the α-synuclein gene, are linked to greatly increasing the risk of Parkinson’s at an early age. This type of PD only affects about 10% of patients.
- Late-onset Parkinson’s disease: The form of PD that develops later in life is due to more common genetic variants that increase susceptibility — combined with specific environmental factors.
Before we dive into Parkinson’s genetic susceptibility, let’s take a look at the environmental factors shown by research to increase Parkinson’s risk. When reading through these environmental factors, note that many of them cause mitochondrial dysfunction. Keep in mind the link to mitochondrial dysfunction in the accumulation of Lewy bodies and α-synuclein.
Environmental Toxins Linked to Parkinson’s
Environmental causes linked to PD include exposure to specific toxicants.[ref] It is likely that these causes are also tied to genetic susceptibilities, such as a decreased ability to detoxify the toxicants. Research shows that these pesticides, herbicides, and industrial solvents increase PD risk:
- Paraquat
- Maneb, Mancozeb
- Organophosphates, chlorpyrifos
- Trichloroethylene
- Heavy metals
Paraquat is a herbicide linked to increasing the risk for PD.[ref] It is still in use in the US, but the EU banned it in 2007. The EPA explains that Paraquat is one of the most widely used herbicides in the US. It is used as a weed and grass killer both in commercial and residential settings.[ref]
Maneb is a fungicide linked to increasing the risk of PD.[ref] It is often used to create Parkinson’s in animal research. Mancozeb is another formulation of Maneb, and it is sold under a variety of brand names. It is used for potato blight, downy mildew on grapes, and other plant fungal diseases.
Trichloroethylene (aka trichlor) is another chemical that is linked to PD.[ref] It is an industrial solvent and is used in refrigerants. Initially, trichloroethylene was used as an anesthetic alternative to ether and chloroform. Better anesthetics have come along, and trichloroethylene is no longer used because it has a nasty side effect of cardiac arrhythmia and neurologic dysfunction. The EPA now lists it as having both carcinogenic and non-carcinogenic health effects. The main route of exposure is contaminated drinking water in areas near industrial spills or landfill leaks.
Twin studies help researchers determine whether a disease is genetic or caused by an environmental factor. The risk of solvent exposure was made clear in a study of 97 twin pairs where one twin had PD and the other didn’t. Exposure to trichloroethylene increased the risk of PD by 6-fold, and combined exposure to perchloroethylene and carbon tetrachloride was also found to increase the risk of PD significantly.[ref]
Organophosphate exposure is also linked to Parkinson’s risk. Chlorpyrifos and other organophosphates increase the risk of Parkinson’s disease, depending on the amount of exposure and the genetic variants the person carries.[ref][ref][ref]
In addition to the risk from pesticide exposure, exposure to heavy metals, including manganese, is linked to an increased risk of Parkinson’s disease. People residing in areas known for metal industries, including ferromanganese plants, or with occupational exposure have higher incidence of Parkinson’s disease and Parkinsonism.[ref]
REM Sleep Disorders and PD:
REM Sleep Behavior Disorder (RBD) is a condition characterized by intense and frequent physical movements during sleep. People with RBD may act out their dreams, such as kicking, punching, or jumping out of bed. They may also experience sleep talking, sleepwalking, and other unusual behaviors while asleep.
Normally during REM sleep, muscles are paralyzed through the activation of two systems: one controlling the input to the spinal cord to prevent muscle movement and the other controlling motor cortex activation.
In people with RBD, researchers think there is an abnormal disinhibition in the pyramidal motor tract. Brain imaging studies show that changes are going on with multiple neurotransmitters, including the cholinergic, noradrenergic, and dopaminergic circuits.[ref]
REM sleep behavior disorder is strongly linked to eventually developing Parkinson’s disease. While initial research showed that about 40% of people with RBD would go on to be diagnosed with Parkinson’s, newer research puts that estimate even higher, with up to 90% eventually developing either Parkinson’s or Lewy-body dementia.[ref]
Importantly, people with RBD have options for treatment and should talk with their doctor and a sleep specialist about determining the cause of RBD and the best options.
Genetic research links mutations in the SCNA and GBA genes (below) to an increased risk of REM sleep behavior disorder.[ref] Notably, other Parkinson-related genes, such as LRRK2, were not linked to RBD, which may indicate more than one pathway is involved in PD.
Circadian Rhythm and Parkinson’s:
Circadian rhythm dysregulation is linked to many of the non-motor symptoms in PD. These issues often occur years before motor-related symptoms (tremors, gait issues) occur. According to animal studies, circadian rhythm disruption directly exacerbates dopaminergic neuronal loss by triggering neuroinflammation.[ref]
Your circadian rhythm – your 24-hour body clock – is set by the increasing and decreasing amounts of two pairs of genes. The CRY and PER genes control what is known as the ‘negative arm’ of the clock, and their levels rise at night. The BMAL1 and CLOCK genes make up the positive arm of the circadian clock, and they are maximized during the day.
These pairs of genes control the expression of thousands of different genes in the body. The sleep-wake cycle is an obvious example of circadian rhythm, but additionally, your hormones, body temperature, neurotransmitter levels, digestive enzymes, detoxification enzymes, and even heart rate are controlled by your circadian rhythm.[ref]
Researchers estimate that 45% of PD patients suffer from depression and anxiety. Sleep rhythm is often disrupted, and the decreased REM sleep is tied to the mood changes in Parkinson’s.[ref]
Animal models of PD show that the circadian rhythm in the substantia nigra is completely gone, and the NLRP3 inflammasome is activated. Significantly, drugs that target specific circadian genes can partly reverse the loss of dopaminergic neurons (in animals).[ref]
Gut microbiome, vitamins, and Parkinson’s
Multiple studies have shown that changes to the gut microbiome are associated with Parkinson’s disease and preceded the condition.[ref][ref]
A new study shed more light on the topic of what is changed in the gut microbiome and why it may impact Parkinson’s risk. The study was a meta-analysis looking at data from multiple gut microbiome studies across different countries.
The results showed that the changes in the gut microbiome affect species of bacteria that produce short-chain fatty acids (acetate, propionate, and butyrate) as well as riboflavin and biotin (B vitamins). The production of vitamins by the gut microbiome is an essential part of how we maintain healthy levels of essential nutrients. The decreased gut production of riboflavin may be particularly important in neurodegeneration and mitochondrial dysfunction in the brain. Biotin may play a role in the immune system response in PD.[ref]
The study also showed a thinning of the mucosal lining. Emulsifiers and surfactants in processed foods can thin the mucosal barrier. Surfactants in medications can also affect the mucosal lining. For more on how this interacts with the immune response, please read: Leak gut genes: Emulsifiers in processed foods
Head trauma, TBI, as a cause?
Head trauma has been suggested as a potential cause of Parkinson’s disease. While traumatic brain injury has been linked to an increased risk of developing the disease, the exact connection is still unclear.
A recent animal study attempted to determine how repetitive mild traumatic brain injury (mTBI) could cause Parkinson’s symptoms. The results showed that repetitive, mild TBI caused aggregation of phosphorylated Tau protein in the substantia nigra of the animals. However, the researchers found mild TBI did not cause fibrils or Lewy body-like alpha-synuclein. The conclusion was that repetitive, mild TBI might add to pre-existing problems with misfolded alpha-synuclein.[ref]
Nicotine: Benefits for preventing Parkinson’s
Cigarette smoking has long been linked in epidemiological studies to reducing the risk of Parkinson’s disease. Of course, smoking is not a good preventative measure since it will likely cause heart disease or lung cancer.
Researchers have been dialing in on why smoking cigarettes seems to reduce the risk of Parkinson’s and have determined it is due to nicotine exposure. They discovered that variants in the synaptic-vesicle glycoprotein 2C (SV2C) impact the degree to which smoking is inversely associated with PD.[ref] [ref]
Let’s dive into the genetic pathways and specific genetic variants that increase the risk of Parkinson’s disease and Lewy body dementia. Keep in mind that these genetic variants do not cause Parkinson’s on their own. Rather, exposure to environmental factors and genetic susceptibility are thought to lead to PD.
Parkinson’s Genotype Report:
Members: Log in to see your data below.
Not a member? Join here.
Why is this section is now only for members? Here’s why…
Lifehacks for Preventing Parkinson’s:
The lifehacks here provide information on what the research shows about PD. The more you know, the better you can make lifestyle and diet decisions.
Related Articles and Topics:
BChE: Nightshade sensitivity, Anesthesia risk, and more
The BCHE gene codes for the butyrylcholinesterase enzyme. The BChE enzyme circulates in the plasma of the blood. It is a cholinesterase that breaks apart choline esters, such as acetylcholine.
GSTs: glutathione-S-transferase enzymes for detoxifying environmental toxins.
Your body has fascinating ways of breaking down and eliminating toxicants, medications, and even hormones made in the body. The glutathione S-transferase genes code for enzymes involved in the removal of a variety of carcinogens and environmental toxins.
Mast cells: MCAS, genetics, and solutions
Mast Cell Activation Syndrome, or MCAS, is a recently recognized disease involving mast cells that misbehave in various ways. Symptoms of MCAS can include abdominal pain, nausea, itching, flushing, hives, headaches, heart palpitations, anxiety, brain fog, and anaphylaxis. Dive into the research on mast cells, genetics, and solutions.
Using your genetic data to solve sleep problems
A good night’s sleep is invaluable – priceless, even – but so many people know the frustration of not being able to sleep well regularly. Not getting enough quality sleep can lead to many chronic diseases, such as diabetes, obesity, dementia, and heart disease. Yes, sleep really is that important!
References:
Bloem, Bastiaan R., et al. “Nonpharmacological Treatments for Patients with Parkinson’s Disease.” Movement Disorders: Official Journal of the Movement Disorder Society, vol. 30, no. 11, Sept. 2015, pp. 1504–20. PubMed, https://doi.org/10.1002/mds.26363.
Bussi, Ivana L., et al. “Involvement of Dopamine Signaling in the Circadian Modulation of Interval Timing.” The European Journal of Neuroscience, vol. 40, no. 1, July 2014, pp. 2299–310. PubMed, https://doi.org/10.1111/ejn.12569.
Chung, C. L., and M. K. Y. Mak. “Effect of Repetitive Transcranial Magnetic Stimulation on Physical Function and Motor Signs in Parkinson’s Disease: A Systematic Review and Meta-Analysis.” Brain Stimulation, vol. 9, no. 4, Aug. 2016, pp. 475–87. PubMed, https://doi.org/10.1016/j.brs.2016.03.017.
Clark, L. N., et al. “Frequency of LRRK2 Mutations in Early- and Late-Onset Parkinson Disease.” Neurology, vol. 67, no. 10, Nov. 2006, pp. 1786–91. PubMed, https://doi.org/10.1212/01.wnl.0000244345.49809.36.
Desplats, Paula, et al. “Combined Exposure to Maneb and Paraquat Alters Transcriptional Regulation of Neurogenesis-Related Genes in Mice Models of Parkinson’s Disease.” Molecular Neurodegeneration, vol. 7, Sept. 2012, p. 49. PubMed, https://doi.org/10.1186/1750-1326-7-49.
Ding, Hongliu, et al. “Association of SNCA with Parkinson: Replication in the Harvard NeuroDiscovery Center Biomarker Study.” Movement Disorders: Official Journal of the Movement Disorder Society, vol. 26, no. 12, Oct. 2011, pp. 2283–86. PubMed, https://doi.org/10.1002/mds.23934.
El Massri, Nabil, et al. “Photobiomodulation-Induced Changes in a Monkey Model of Parkinson’s Disease: Changes in Tyrosine Hydroxylase Cells and GDNF Expression in the Striatum.” Experimental Brain Research, vol. 235, no. 6, June 2017, pp. 1861–74. PubMed, https://doi.org/10.1007/s00221-017-4937-0.
Fitzmaurice, Arthur G., et al. “Aldehyde Dehydrogenase Variation Enhances Effect of Pesticides Associated with Parkinson Disease.” Neurology, vol. 82, no. 5, Feb. 2014, pp. 419–26. PubMed Central, https://doi.org/10.1212/WNL.0000000000000083.
Fleming, Sheila M. “Mechanisms of Gene-Environment Interactions in Parkinson’s Disease.” Current Environmental Health Reports, vol. 4, no. 2, June 2017, pp. 192–99. PubMed, https://doi.org/10.1007/s40572-017-0143-2.
Goldman, Samuel M., et al. “Solvent Exposures and Parkinson Disease Risk in Twins.” Annals of Neurology, vol. 71, no. 6, June 2012, pp. 776–84. PubMed, https://doi.org/10.1002/ana.22629.
Goldwurm, S., et al. “Evaluation of LRRK2 G2019S Penetrance: Relevance for Genetic Counseling in Parkinson Disease.” Neurology, vol. 68, no. 14, Apr. 2007, pp. 1141–43. PubMed, https://doi.org/10.1212/01.wnl.0000254483.19854.ef.
Gu, Zhuqin, et al. “Association of ARNTL and PER1 Genes with Parkinson’s Disease: A Case-Control Study of Han Chinese.” Scientific Reports, vol. 5, Oct. 2015, p. 15891. PubMed Central, https://doi.org/10.1038/srep15891.
Hamblin, Michael R. “Shining Light on the Head: Photobiomodulation for Brain Disorders.” BBA Clinical, vol. 6, Oct. 2016, pp. 113–24. PubMed Central, https://doi.org/10.1016/j.bbacli.2016.09.002.
Hamilton, Catherine, et al. “Exploring the Use of Transcranial Photobiomodulation in Parkinson’s Disease Patients.” Neural Regeneration Research, vol. 13, no. 10, Oct. 2018, pp. 1738–40. PubMed Central, https://doi.org/10.4103/1673-5374.238613.
Huang, Yongpan, et al. “The Association between E326K of GBA and the Risk of Parkinson’s Disease.” Parkinson’s Disease, vol. 2018, 2018, p. 1048084. PubMed, https://doi.org/10.1155/2018/1048084.
International Journal of Clinical and Experimental Pathology. https://e-century.us/web/journal.php?journal=ijcep. Accessed 13 May 2022.
Jensen, Bente Rona, et al. “Effects of Long-Term Treatment with T-PEMF on Forearm Muscle Activation and Motor Function in Parkinson’s Disease.” Case Reports in Neurology, vol. 10, no. 2, Aug. 2018, pp. 242–51. PubMed Central, https://doi.org/10.1159/000492486.
Johnstone, Daniel M., et al. “Turning On Lights to Stop Neurodegeneration: The Potential of Near Infrared Light Therapy in Alzheimer’s and Parkinson’s Disease.” Frontiers in Neuroscience, vol. 9, Jan. 2016, p. 500. PubMed Central, https://doi.org/10.3389/fnins.2015.00500.
Kim, Jong-Min, et al. “The LRRK2 G2385R Variant Is a Risk Factor for Sporadic Parkinson’s Disease in the Korean Population.” Parkinsonism & Related Disorders, vol. 16, no. 2, Feb. 2010, pp. 85–88. PubMed, https://doi.org/10.1016/j.parkreldis.2009.10.004.
Li, Siyue, et al. “A New Perspective for Parkinson’s Disease: Circadian Rhythm.” Neuroscience Bulletin, vol. 33, no. 1, Dec. 2016, pp. 62–72. PubMed Central, https://doi.org/10.1007/s12264-016-0089-7.
Liu, Mei, et al. “Trichloroethylene and Parkinson’s Disease: Risk Assessment.” Molecular Neurobiology, vol. 55, no. 7, July 2018, pp. 6201–14. PubMed, https://doi.org/10.1007/s12035-017-0830-x.
Malling, Anne Sofie Bøgh, et al. “Effect of Transcranial Pulsed Electromagnetic Fields (T-PEMF) on Functional Rate of Force Development and Movement Speed in Persons with Parkinson’s Disease: A Randomized Clinical Trial.” PloS One, vol. 13, no. 9, 2018, p. e0204478. PubMed, https://doi.org/10.1371/journal.pone.0204478.
Mischley, Laurie K., et al. “Role of Diet and Nutritional Supplements in Parkinson’s Disease Progression.” Oxidative Medicine and Cellular Longevity, vol. 2017, 2017, p. 6405278. PubMed, https://doi.org/10.1155/2017/6405278.
Mitsui, Jun, et al. “Mutations for Gaucher Disease Confer High Susceptibility to Parkinson Disease.” Archives of Neurology, vol. 66, no. 5, May 2009, pp. 571–76. PubMed, https://doi.org/10.1001/archneurol.2009.72.
Morberg, B. M., et al. “Effects of Transcranial Pulsed Electromagnetic Field Stimulation on Quality of Life in Parkinson’s Disease.” European Journal of Neurology, vol. 25, no. 7, July 2018, pp. 963-e74. PubMed, https://doi.org/10.1111/ene.13637.
PDGene. http://www.pdgene.org/view?poly=rs356218. Accessed 13 May 2022.
Ross, Owen A., et al. “LRRK2 Exonic Variants and Susceptibility to Parkinson’s Disease.” Lancet Neurology, vol. 10, no. 10, Oct. 2011, pp. 898–908. PubMed Central, https://doi.org/10.1016/S1474-4422(11)70175-2.
Satake, Wataru, et al. “Genome-Wide Association Study Identifies Common Variants at Four Loci as Genetic Risk Factors for Parkinson’s Disease.” Nature Genetics, vol. 41, no. 12, Dec. 2009, pp. 1303–07. PubMed, https://doi.org/10.1038/ng.485.
Tan, Eng-King, et al. “Multiple LRRK2 Variants Modulate Risk of Parkinson Disease: A Chinese Multicenter Study.” Human Mutation, vol. 31, no. 5, May 2010, pp. 561–68. PubMed, https://doi.org/10.1002/humu.21225.
Videnovic, Aleksandar, and Diego Golombek. “Circadian Dysregulation in Parkinson’s Disease.” Neurobiology of Sleep and Circadian Rhythms, vol. 2, Nov. 2016, pp. 53–58. PubMed Central, https://doi.org/10.1016/j.nbscr.2016.11.001.
Walton, Courtney C., et al. “Cognitive Training for Freezing of Gait in Parkinson’s Disease: A Randomized Controlled Trial.” NPJ Parkinson’s Disease, vol. 4, 2018, p. 15. PubMed, https://doi.org/10.1038/s41531-018-0052-6.
Wang, Youpei, et al. “Glucocerebrosidase L444P Mutation Confers Genetic Risk for Parkinson’s Disease in Central China.” Behavioral and Brain Functions: BBF, vol. 8, Dec. 2012, p. 57. PubMed, https://doi.org/10.1186/1744-9081-8-57.
“What Is Parkinson’s?” Parkinson’s Foundation, https://www.parkinson.org/understanding-parkinsons/what-is-parkinsons. Accessed 13 May 2022.
Yu, Rwei-Ling, et al. “Aldehyde Dehydrogenase 2 Is Associated with Cognitive Functions in Patients with Parkinson’s Disease.” Scientific Reports, vol. 6, no. 1, July 2016, p. 30424. www.nature.com, https://doi.org/10.1038/srep30424.
.