Key takeaways:
~ Glutamate is the main excitatory neurotransmitter in the brain and central nervous system.
~ Levels of glutamate in the synapse are controlled by glutamate transporters, including EAAT2 and EAAT1.
~ Glutamate receptors, including AMPA, NMDA, and kainate receptors, regulate synaptic transmission and neuronal plasticity.
~ Research connects glutamate imbalance to conditions like ALS, long COVID, ADHD, schizophrenia, and neuroinflammation.
~ Genetic variants in the glutamate transporter and glutamate receptors can increase your risk for glutamate imbalances in the brain.
What is glutamate and how is it regulated?
Glutamate is the primary excitatory neurotransmitter in the central nervous system (brain, spinal cord). Glutamate also plays a minor role in nerve signaling in the peripheral nervous system.[ref]
A neuron sends a message through an electrical impulse that causes the release of a chemical messenger from the axon. In this case, the messenger is glutamate.
Glutamate is released from the axon of one neuron into the synapse and is received by glutamate receptors in the adjacent neuron, thus sending a signal from one nerve to the next. Nearby glial cells, mainly astrocytes, control the amount of glutamate that remains in the synapse. Maintaining optimal levels of glutamate in the synaptic space is important. Low levels can lead to low energy, while excess glutamate can lead to cell death in the neurons.[ref]

In this article, I’ll look at how glutamate levels are controlled by glutamate transporters and how the glutamate signal is received by glutamate receptors. This is a continuation of my article on how glutamate is synthesized.
Glutamate transporters: Taking up glutamate from the synapse
Glutamate transporters regulate how much glutamate is available in the synapse, thereby controlling the level of extracellular glutamate that can activate nearby neurons. The glutamate transporters are found on nearby glial cells, called astrocytes, and on the axons of neurons. Astrocytes are a non-neuronal cell in the brain that helps neurons function. They have glutamate receptors which take up excess glutamate in the synapse as a way to regulate the signaling process. [ref]

Basically, you don’t want too much glutamate in the synapse because it’s an excitatory neurotransmitter. As an extreme example, too much glutamate is a cause of seizures. Too much glutamate can be toxic to neurons and cause neuronal death.
On the other hand, a lack of glutamate causes problems with focusing, impairs memory, and causes low energy.
Balance is key with glutamate, and the glutamate transporters on astrocytes are essential here.
Glutamate is directly or indirectly involved in almost all brain functions, and the different glutamate transporters control glutamate levels in different ways.
There are five transporters for glutamate:[ref]
- EAAT1 and EAAT2 are the primary transporters in the brain and spinal cord that regulate glutamate levels there.
- EAAT3 is also found in certain brain regions and may play a role in glutamate levels under specific circumstances. It is also found in the kidneys, liver, heart, and intestinal mucosa.[ref]
- EAAT4 is localized to Purkinje cells
- EAAT5 is mainly in the retina and is involved in the way that rods and cones transmit vision. [ref]
EAAT stands for excitatory amino acid transporter. While the EAAT transporters primarily move the amino acid glutamate into astrocytes, they can also transport aspartate, another amino acid.
While we think of this as a one-way street with glutamate being released from a neuron’s axon into the synapse and then taken up either by the nearby neuron or by an astrocyte, this system can actually work as a two-way street. “Glutamate transporters can also run in reverse, resulting in glutamate release from cells.”[ref]
Let’s dig into details on two of the primary glutamate transporters, EAAT2 and EAAT1…
Glutamate transporter-1 (EAAT2, GLT-1): Controlling the glutamate in the synapse
Glutamate transporter-1 (GLT-1) is a transporter that regulates extracellular glutamate levels in the central nervous system. It is called EAAT2 in humans (GLT-1 in mice) and is encoded by the SLC1A2 gene.
EAAT2 is a sodium- and potassium-dependent transporter that moves sodium ions into the cell along with glutamate while balancing this with potassium moving out of the cell. Chloride and other ions also flow in and out of the cells via EAAT2.[ref]
Glutamate is constantly being released into the synapse, and inhibiting glutamate uptake causes it to build up within seconds.[ref]
EAAT2 is responsible for the majority of glutamate uptake, with animal studies showing that it is responsible for moving around 40% of the glutamate from the synapse. When researchers knock out the EAAT2 receptors from astrocytes, it causes seizures that are eventually lethal in animals.[ref] EAAT2 is primarily expressed in astrocytes, which are a type of glial cell that supports neurons and helps the brain function, and excitatory neurons. [ref] Glutamate is then recycled back to the neuron as glutamine.
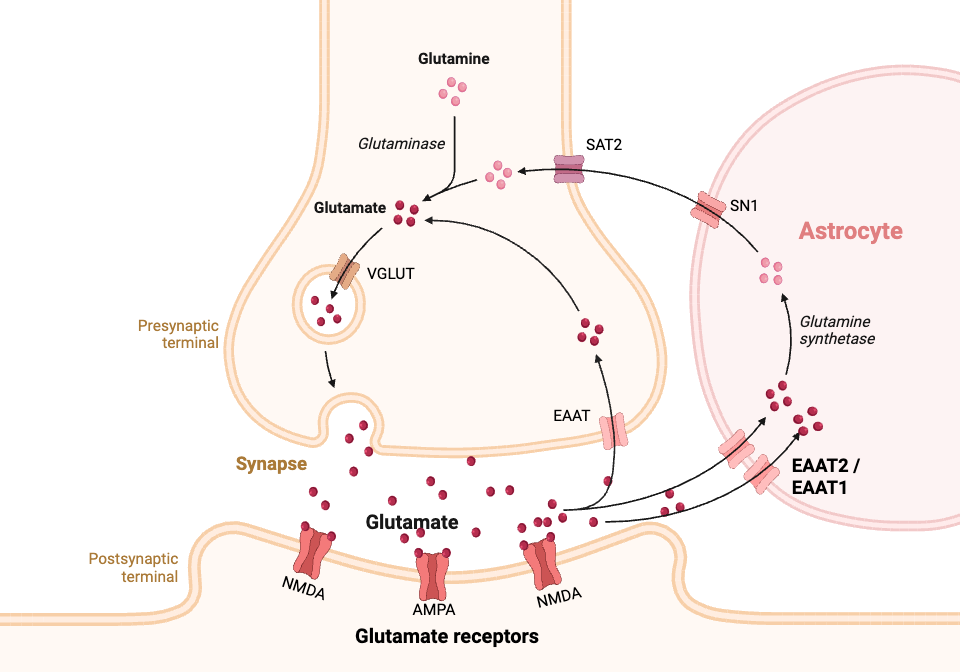
Glutamate aspartate transporter (EAAT1):
The glutamate aspartate transporter (GLAST) is referred to as EAAT1 and is encoded by the SLC1A3 gene.
EAAT1 is a sodium-potassium transporter that moves glutamate, aspartate, and sodium into a cell while also moving potassium out of the cell. It is primarily expressed in astrocytes in the cerebellum and neocortex regions of the brain.[ref]
While EAAT2 is the main way that glutamate is removed from the synapse, EAAT1 also plays a role in preventing excitotoxicity. When EAAT1 (SLC1A3) is knocked out in mice, they are more susceptible to neuronal death from concussions and also are susceptible to retinal degeneration. In addition, the lack of EAAT2 causes mice to have abnormal social behavior and poor nesting behavior.[ref][ref]
Rare mutations in SLC1A3 cause a high risk of glaucoma, pointing to the role of glutamate regulation in the optic nerve.[ref] An SLC1A3 variant found in about one percent of the population increases the risk of Tourette syndrome, indicating that glutamate regulation via EAAT1 is important in Tourette’s.[ref] Other rare mutations in EAAT1 cause episodic ataxia and epilepsy. Note that there aren’t many common polymorphisms in EAAT1, indicating that variants aren’t tolerated in this important gene.
xCT-system: exchanging glutamate for cysteine
Another factor to consider with glutamate regulation in the brain is that it is involved in glutathione production. Glutathione is the primary intracellular antioxidant in the brain, and glutamate is required for its synthesis, along with cysteine and glycine. Cysteine is usually the limiting factor in glutathione production.[ref]
Related article: Glutathione production & related genetic variants
Interestingly, the transporter that moves cystine into cells is the xCT transporter, which simultaneously moves glutamate out of the cell when it moves cystine in. (Cystine is the oxidized form of cysteine.)
The xCT antiporter consists of two subunits that are encoded by the SLC7A11 and SLC3A2 genes. SLC7A11 expression is dependent on Nrf2, which is a transcription factor that is upregulated in response to stress.
Interestingly, tBHQ, which is a compound added to foods and cosmetics as a preservative, induces Nrf2 and “robustly increase xCT protein levels”.[ref] Considering that increasing xCT can cause more glutamate in the synapse, it makes sense that this preservative is linked to ADHD and hyperactivity in some children.[ref]
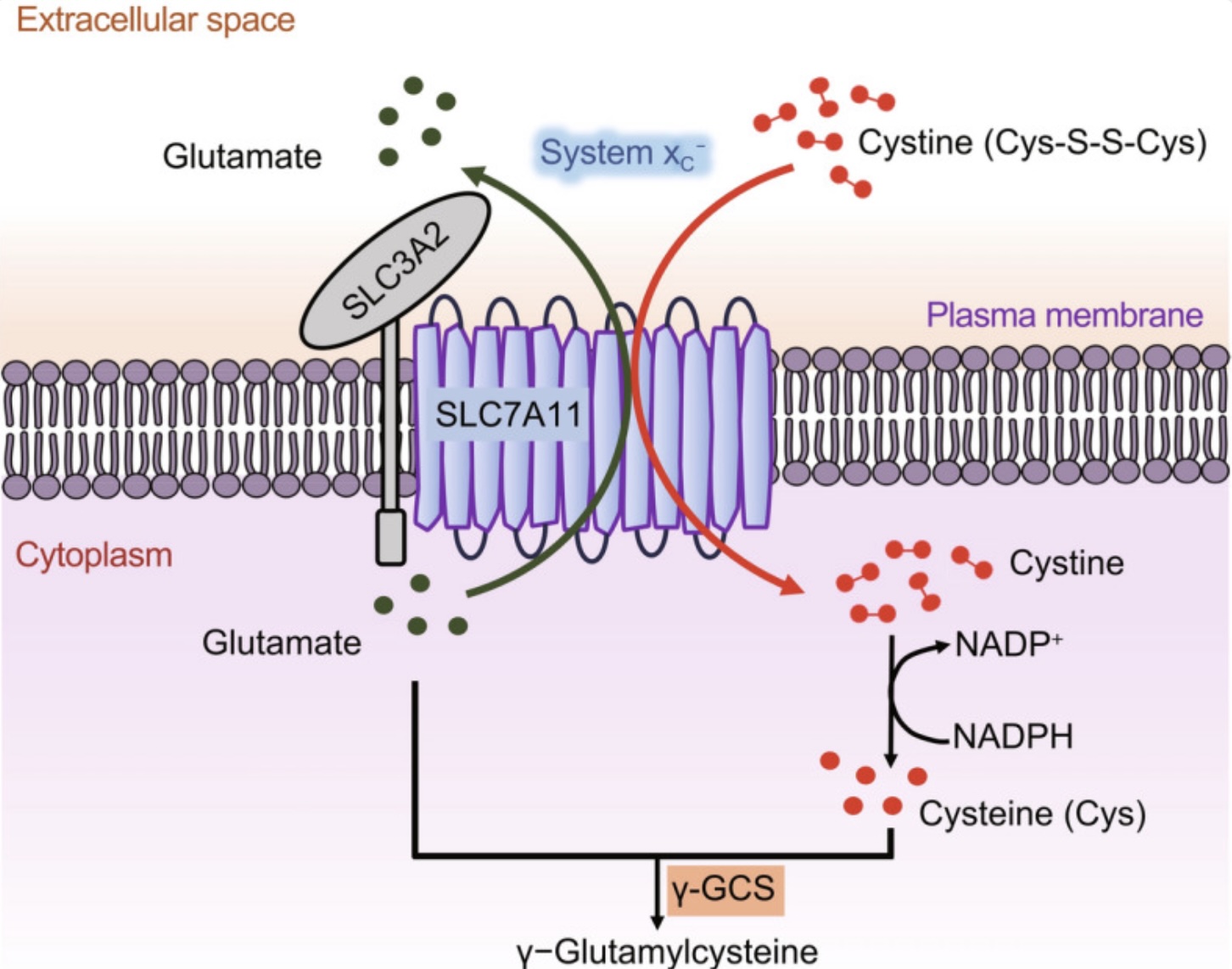
Regulation of Glutamate Transporter Levels:
The availability of glutamate transporters in astrocytes and other glial cells depends on a number of different factors.
Genetic variants (SNPs) can alter the function of the transporter. These are discussed in the genotype report below.
Your circadian rhythm also strongly affects EAAT2 levels in the brain. The expression of EAAT2 is higher in the morning and should drop to its low at night. This changing level of EAAT expression alters glutamate levels in the brain over the course of a day. It is one of the reasons that blood pressure changes from morning to night. Animal studies show that the core circadian rhythm genes control the changes to glutamate transporter during the day, and dysregulation of these genes causes changes to the glutamate rhythm.[ref][ref][ref]
Post-translation regulations occur in several ways to modulate EAAT levels, and microRNAs also affect these glutamate transporter levels. Let’s look at both in more detail…
Post-translational regulation of Glutamate transporters:
Post-translational modifications and epigenetic regulation also significantly affect the number of glutamate transporters available. Post-translational modifications of EAAT1 and EAAT2 include palmitoylation, sumoylation, s-nitrosylation, and ubiquitination.[ref]
Palmitoylation is a modification that adds a fatty acid called palmitate to the glutamate transported so that it can be anchored in the cell membrane of the astrocyte. When palmitoylation is inhibited, the EAAT2 transporter is impaired and there is less glutamate taken up into the astrocytes resulting in glutamate toxicity in the synapse.[ref]
↓ Palmitoylation = ↓ EAAT2 on cell membrane = ↑ glutamate toxicity
Sumoylation is the process by which a protein called SUMO is attached to the glutamate transporter, which results in it being localized inside the cell instead of being available on the cell membrane to take up glutamate from the synapse. Too much sumoylation is thought to be one cause of ALS.[ref] Increasing sumoylation increases EAAT2 on the cell membrane of the astrocyte causing more glutamate to be taken from the synapse into the astrocyte.[ref]
↑ Sumoylation = ↓ EAAT2 on cell membrane = ↑ glutamate toxicity
S-nitrosylation is the process of attaching a nitroso group (-NO) to a molecule. S-nitrosylation of EAAT2 causes a decrease in transporter uptake of glutamate and an increase of glutamate in the synapse. This may play a role in Alzheimer’s disease with the excitotoxicity damaging neurons. Exposure to air pollution causing excess reactive nitrogen species increases the risk of Alzheimer’s.[ref][ref]
↑ S-nitrosylation = ↓ EAAT2 on cell membrane = ↑ glutamate toxicity
Ubiquitination is a process that attaches ubiquitin to a protein to a molecule. In the case of EAAT2, ubiquitination signals for the transporter to be kept in the cytosol of the astrocyte rather than being located on the cell membrane to take up glutamate. This can be reversed by deubiquitination in some instances.[ref]
↑ Ubiquitination = ↓ EAAT2 on cell membrane = ↑ glutamate toxicity
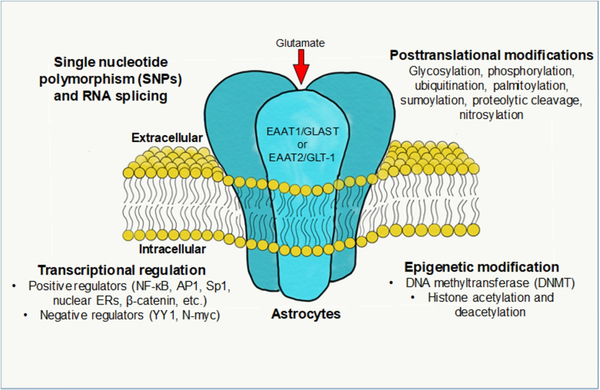
MiRNAs and post-transcriptional regulation of glutamate transporters:
MicroRNAs are short strands of RNA that can bind to mRNA to prevent it from being translated into its protein. In this case, several different miRNAs are capable of binding to SLC1A2, SLC1A3, and other related mRNAs – preventing them from being translated into the transporter.
This type of post-transcriptional regulation is a fast way for the cell to respond to changes in the cell environment — turning up or down gene expression quickly.
One example is miR-124, which is a microRNA expressed in neuronal cells that accounts for 25–48% of total miRNA in the adult brain. It is upregulated during development and also during adult neurogenesis. Studies show that miR-124 is downregulated in neurodegenerative disorders, such as Alzheimer’s, ALS, and Parkinson’s.[ref] Increasing miR-124 in astrocytes of a mouse model of ALS shows that it prevents the loss of EAAT2 transporters and thus balances out glutamate.[ref]
miR-218 is another miRNA that regulates EAAT2 expression. In astrocytes, miR-218 downregulates EAAT2 expression. Similarly, miR-30 upregulation causes lower EAAT2 expression in a model of Parkinson’s disease.[ref]
I’m just hitting a few highlights here as examples, and there are more miRNAs involved in glutamate regulation.
Related article: MicroRNAs
Glutamate transporters and neurological diseases
Reduced expression of glutamate transporters allows higher glutamate levels to remain in the synapse, and excess glutamate is excitatory and can be neurotoxic at high levels. This can have myriad negative effects on the way that the brain functions.
ME/CFS and long Covid:
A recent study showed that glutamate and aspartate levels are significantly higher in the brains of individuals with ME/CFS or long Covid when compared to a healthy control group.[ref]
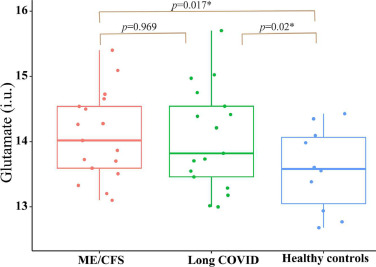
Related article: ME/CFS genetic connections
Amyotrophic lateral sclerosis (ALS, Lou Gherigs disease):
The EAAT2 transporter is reduced in the spinal cord and motor cortex of people with ALS. This results in higher glutamate levels in the cerebrospinal fluid. Research points to the EAAT2 protein being cleaved by caspase-3 as a driving factor in the loss of motor neurons in ALS.[ref] Animal models of ALS show that EAAT2 loss of function precedes the loss of neurons and the onset of disease.[ref]
Alzheimer’s and Parkinson’s:
Glutamatergic neurons are lost in Alzheimer’s disease, and there is a reduction in glutamate transport in the brain. [ref] The rare mutations that cause early-onset Alzheimer’s cause the astrocytes to have reduced expression of EAAT2.[ref] The rare mutations in the LRRK2 gene that cause familial Parkinson’s also interact with EAAT2 expression in glial cells.[ref]
Related article: APOE and Alzheimer’s disease | Parkinson’s genetic susceptibility
Epilepsy:
The glutamate transporters are responsible for keeping glutamate low, and thus they play an important role in epilepsy. Increasing EAAT2 shows promise for controlling spontaneous recurrent seizures.[ref]
ADHD:
Studies involving adults with ADHD show that glutamate and GABA concentrations are similar to healthy controls when at rest, but when performing tasks that required attention control, there was a smaller GABA increase in the ADHD group. GABA is the inhibitory neurotransmitter that is the “off” switch for excitatory neurons.[ref]
Related article: ADHD genes
Post-operative neuroinflammation:
Surgery or trauma causes the release of substances that can cause an inflammatory response in the brain. This can result in neurocognitive disorders or brain fog after surgery. Recent research shows that neuroinflammation causes changes in glial cells that then decrease the uptake of glutamate (leaving more of the excitatory neurotransmitter in the synapse).[ref]
Depression with inflammation:
In a study on teens with depression, researchers found that higher IL-6 levels correlated with higher glutamate levels in the anterior cingulate cortex.[ref]
Other conditions linked to lower EAAT activity include:
- Anxiety and depression[ref]
- Chronic pain[ref]
- Migraines[ref]
- Mercury exposure[ref]
- Cytomegalovirus[ref]
Schizophrenia and higher EAAT:
The EAAT1 and EAAT2 glutamate transporter levels are increased in specific brain regions in people with schizophrenia compared to people without schizophrenia.[ref]
Related article: Schizophrenia genetics and environmental factors
Glutamate receptors:
Let’s switch gears and talk about how glutamate receptors on neurons propagate the neuronal signal. Glutamate receptors receive the signal from glutamate released by a nearby neuron, transmitting a signal to the next neuron to fire.
Similar to the glutamate transporters above, glutamate receptors are primarily located on the cell membrane of the neuron and bind to glutamate from the synapse. However, the availability of the glutamate receptors is a dynamic process and they can be moved into the cytosol of the cell and inactivated.[ref][ref]
The glutamate receptors are also essential for synaptic plasticity, which is how the brain adapts and modifies connections in learning and memory formation.
There are two main categories of glutamate receptors:
- ionotropic glutamate receptors
- metabotropic glutamate receptors
Ionotropic Glutamate Receptors (iGluRs)
Ionotropic glutamate receptors are ion channels that are induced by glutamate to signal neuronal transmission throughout the brain and central nervous system. The binding of glutamate to these ion channels causes fast, excitatory neurotransmission. Glutamate from the synapse binds to the receptor and causes an influx of ions (Na+, K+, and sometimes Ca2+) into the cell.[ref][ref]
There are three types of ionotropic glutamate receptors:
- AMPA receptors
- NMDA receptors
- Kainate receptors
1) AMPA Receptors (GluA1-4):
The AMPA receptor is encoded by the GRIA1- 4 genes, with multiple proteins being assembled together in the ER to make up the receptor. Studies refer to these parts of the receptor as GluA1 – GluA4.
AMPA receptors are the most prevalent glutamate receptors. Glutamate binds to this receptor to allow Na⁺/K⁺ to enter the neuron. This causes basal synaptic transmission.[ref]
Rare genetic mutations in the GRIA1 gene are associated with intellectual disability and neurological disorders.[ref] Similarly, mutations that cause gain or loss of function in GRIA3 lead to neurodevelopmental disorders and seizures.[ref]
Interestingly, AMPA receptors and glutamate are involved in the release of reproductive hormones from the brain and these receptors control ovulation in women.[ref]
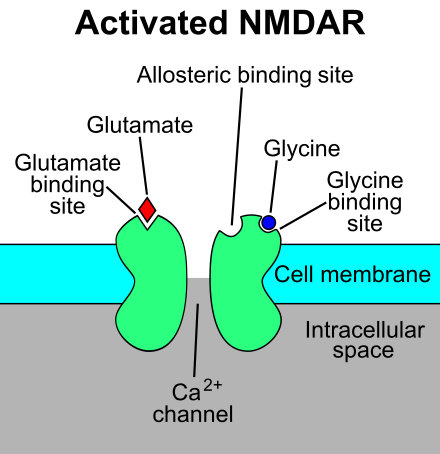
Weak glutamate stimulation will cause the activation of AMPA receptors, while NMDA receptors require a strong stimulus. Both types of receptors are found on the cell membrane of glutamatergic neurons.[ref]
2) NMDA Receptors (GluN1, GluN2A-D, GluN3A/B):
The NMDA (N-Methyl-d-aspartate) receptor is also assembled from multiple proteins. It requires both glycine and glutamate to bind to it simultaneously for activation.[ref]
Glutamate binds to the NMDA receptor allowing calcium ions to flow into the neuron. This binding is controlled by magnesium so that the calcium ions only flow in at the right time for the neuron to fire. Essentially, magnesium ions block the receptor, restricting entry of calcium ions, when the neuron is activated. When the magnesium block is removed, the neuron is ready to fire again. I’m probably not explaining that very well. [ref]
The NMDA receptor is named as such because it is the only one of the glutamate receptors that NMDA will bind to. NMDA is an aspartate derivative that mimics glutamate when binding to the NMDA receptor.
Substances that can block the NMDA receptor (NMDA antagonists) include:[ref]
- ketamine
- amantadine (Parkinson’s drug) and memantadine (Alzheimer’s)
- dextromethorphan (cough syrup)
- angel dust (street drug)
- riluzole (ALS medication)
- methoxetamine (hallucinogenic drug)
- kynurenic acid (tryptophan metabolite)
- methadone (also an opioid receptor antagonist)
- tramadol (pain, anesthesia)
Blocking the NMDA receptor can act as an anesthesia and also induce a dissociative state, paranoid delusions, confusion, agitation, and mood alterations. Repeatedly blocking the NMDA receptor can cause excess glutamate in the synapse and neurotoxicity (NMDA receptor antagonist neurotoxicity, NAT). Medications are compounded in a way to avoid this, but neurotoxicity is possible with drug abuse.
Related articles: Tryptophan Metabolism and kynurenic acid
3) Kainate Receptors (GluK1-5):
The kainate receptors are found throughout the central nervous system where they modulate the amount of glutamate in the synapse. They can act both to release glutamate into the synapse and can take up glutamate out of the synapse. One place where kainate receptors play an important role is in the olfactory bulb. There they interact with zinc to influence odor detection. Kainate receptors are also important in the ear for modulating the transmission of sounds via the tiny hairs in the cochlea.[ref][ref]
Genetic variants in kainate receptors are associated with alcohol use, epilepsy, autism, migraines, and schizophrenia.[ref]
Kainate receptors are named as such because, in addition to glutamate, they also can strongly respond to kainate. Kainate, also known as kainic acid, is an amino acid that activates glutamate receptors in the brain. It was first isolated from seaweed in the 1950s and is used in epilepsy research because it activates the kainate receptor.
Metabotropic Glutamate Receptors (mGluRs):
The metabotropic glutamate receptors (abbreviated mGluRs) are G-protein-coupled receptors found in several regions of the brain. They are involved in learning, memory, anxiety, and pain perception. The mGluRs essentially fine-tune the synaptic transmission in the glutaminergic neurons.[ref]
There are eight different metabotropic glutamate receptors and some of them increase excitability while others turn down the glutamate release to regulate neuroinflammation.
The synaptic function of several of the metabotropic glutamate receptors is altered in autism spectrum disorder, and glutamatergic neurons are important here. The metabotropic glutamate receptors have been identified in multiple studies as playing a role in ASD traits.
In children with autism spectrum disorder, researchers have found that there is a significant decrease in mRNA levels of metabotropic glutamate receptors – specifically GRM5, GRM6, and GRM7. Genetic variants in these genes are statistically linked to an increased relative risk of autism.[ref]
Inflammation and glutamatergic function:
IL-1B is a proinflammatory cytokine that is upregulated in response to infection or other inflammatory stimuli. IL-1B can act on the NMDA receptors to increase function. It also can inhibit the uptake of glutamate by astrocytes.[ref]
TNF-alpha is another proinflammatory cytokine that can act on a glutamate receptor – in this case, AMPA receptors. It also can increase presynaptic glutamate release in neurons and decrease the glutamate transporter activity, leading to neuronal excitotoxicity at high enough levels. [ref]
Related article: TNF-alpha: Genetic variants that increase inflammation
Inflammation in the brain has differing effects on glutamate levels, depending on whether it is acute inflammation that resolves vs. chronic inflammation.
Astrocytes express receptors for multiple immune-related molecules. When they are activated acutely, such as during an injury, stroke, or infection, astrocytes can act as immune system cells and release inflammatory mediators. In this acute inflammatory state, there is impaired glutamate clearance which can cause excitotoxicity. [ref]
Chronic activation, on the other hand, causes astrocytes to differentiate and proliferate, which is called reactive astrocytosis. In this state, they can promote healing after a stroke or trauma. However, when activated by a pathogen, astrocytes can fail to perform normal functions, such as clearing glutamate from the synapse.[ref]
Let’s switch gears now and look at some of the genetic variants in the glutamate transporters and receptors.
Genotype report:
Lifehacks:
Let’s take a look first at substances that interact with the glutamate transporters. As a reminder, substances that decrease glutamate transporters cause increased glutamate in the synapse which can be neurotoxic at higher levels.
Please talk with your doctor if you are on any medications or have any questions at all — before you try supplements that could affect glutamate levels.
Glutamate transporter (EAAT2, EAAT2) interactions:
I’m going to start off with compounds and environmental factors that interact with glutamate transporters. Keep in mind that reduced glutamate uptake by EAAT2 causes more glutamate to remain in the synapse. This can cause neurotoxicity if the glutamate level remains too high.
Drugs that affect EAAT2 expression:
Clavulanic acid increases EAAT2 which then causes less glutamate in the synapse. Clavulanic acid plus amoxicillin is known as amoxiclav (Augmentin) and is commonly used as an antibiotic.[ref]
Dexamethasone, a corticosteroid used for inflammation and autoimmune conditions, induces EAAT2 in astrocytes in the cortex.[ref]
Amitriptyline, a tricyclic antidepressant, upregulates both EAAT2 and EAAT1 in animal studies.[ref]
Ceftriaxone (an antibiotic) increases EAAT2 expression in some animal studies, but not all studies agree. In human cell lines, ceftriaxone activates NF-κB, which then causes an increase in EAAT2 gene expression.[ref]
Thiopyridazine derivatives are antiplatelet drugs (e.g. Plavix) that are converted into the prodrug form in the liver using CYP2C19. Studies show that they increase EAAT2 expression.[ref]
Natural substances that increase glutamate transporters:
Harmine, which is a naturally occurring alkaloid found in Syrian rue and ayahuasca, increases EAAT2 expression and thus decreases glutamate excitotoxicity. However, it is also an MAOA inhibitor.[ref] Lemon balm contains low levels of harmine.[ref] Interestingly, there’s a clinical trial using lemon balm (melissa officinalis) extract for Alzheimer’s that had positive effects.[ref]
Substances that decrease glutamate transporters:
Nicotine: Exposure to nicotine from e-cigarettes causes downregulation of EAAT2 after 3 months of use (in mice).[ref] The increase in glutamate in the synapse is thought to be involved in nicotine addiction as well as the stimulatory effect.[ref]
Aspartate, an amino acid, competes with glutamate in binding to EAAT. Researchers use an aspartate analog to mimic EAAT2 inhibition.[ref] Note that aspartame (artificial sweetener) contains aspartate plus phenylalanine. Some dietary supplements, such as magnesium aspartate, also contain aspartate.
Manganese, at higher levels, causes the glutamate transporters to be localized into the cytosol, resulting in higher glutamate levels in the synapse.[ref]
Related article: Manganese, genetics, diet, and supplements
Kainic acid is another EAAT2 inhibitor that increases glutamate in the synapse.[ref] Kainic acid is found in nature only in certain types of seaweed and is unlikely to be eaten.
Rapamycin was shown in a mouse model of Parkinson’s to preserve glutamate transporter function, keeping glutamate at the right levels.[ref]
Glutamate receptors:
The availability of glutamate receptors is the other half of the picture for both stimulation of the neurons and good brain function – as well as for removing glutamate from the synapse.
Excess activation of the glutamate receptors, specifically NMDA receptors, causes excitotoxicity from too much neuronal stimulation.[ref]
Substances that act on glutamate receptors:
This is just hitting the highlights and isn’t exhaustive.
Ketamine:
Ketamine is a non-competitive NMDA receptor antagonist. It has fast-acting antidepressive effects, and animal studies show that the effects on behavior last well beyond the half-life. [ref]
Dextromethorphan:
Commonly found in cough syrups, dextromethorphan is a low-affinity uncompetitive NMDAR antagonist. It also acts on nicotinic receptors. Studies on it show that can reverse pain sensation in peripheral and central neurons.[ref]
Aniracetam:
Aniracetam enhances AMPA receptor function as a positive allosteric modulator.[ref]
Ashwagandha:
Studies show that components of ashwagandha, a commonly used herbal supplement, act to reduce NMDA receptor expression.[ref][ref]
Related article: Ashwagandha research studies
Ginkgo biloba:
Bilobalide, a molecule in Ginko biloba, has been shown in studies to reduce the expression of the NMDA receptor.[ref][ref]
Dietary interactions with glutamate receptors:
Aspartame:
Aspartame contains aspartate that can compete with and prevent glutamate from binding to the NMDA receptor. This causes more glutamate to remain in the synapse.[ref]
Animal studies show that aspartame causes anxiety-like behavior and learning and memory deficits. These behavior changes are actually heritable and affect the offspring. The studies involve using an equivalent amount of aspartame as found in regularly drinking one 16-oz. diet soda per day.[ref]
Decanoic acid:
A medium-chain triglyceride found in coconut oil, decanoic acid inhibits AMPA receptors at high concentrations. This is likely why MCT oil has antiseizure actions.[ref]
Balancing glutamate receptors and transporters:
Vitamin B6:
Converts glutamate into GABA, which is an inhibitory neurotransmitter. A deficiency of vitamin B6 can cause an imbalance of glutamate to GABA — too much of the excitatory neurotransmitter and not enough of the inhibitory neurotransmitter.
Genetic variants can influence how much vitamin B6 you need and whether you are likely to respond better to the active P5P form. Read the full article on B6 here.
Gene | RS ID | Effect Allele | Your Genotype | Notes About Effect Allele |
---|---|---|---|---|
ALPL | rs1256335 | G | -- | Decreased vitamin B6 |
ALPL | rs1697421 | T | -- | Slightly decreased vitamin B6 levels |
ALPL | rs1780316 | T | -- | Slightly decreased vitamin B6 levels |
NBPF3 | rs4654748 | C | -- | Slightly decreased vitamin B6 levels |
ALDH7A1 | rs121912707 | G | -- | Mutation linked to vitamin B6 dependent epilepsy (rare) |
ALDH7A1 | rs121912708 | A | -- | rare mutation linked to vitamin B6 dependent epilepsy |
PDXK | rs2010795 | A | -- | increased relative risk of Parkinson’s disease |
PNPO | rs17679445 | A | -- | 40% reduced catalytic activity and impaired binding |
PNPO | rs370243877 | T | -- | carrier of a pathogenic mutation in the PNPO gene |
PNPO | rs550423482 | A | -- | carrier of a pathogenic mutation in the PNPO gene |
PNPO | rs773450573 | A | -- | carrier of a pathogenic mutation in the PNPO gene |
PNPO | rs796052869 | T | -- | carrier of a pathogenic mutation in the PNPO gene |
PNPO | rs796052870 | C | -- | carrier of a pathogenic mutation in the PNPO gene |
Glycine:
Glycine is essential for the activation of the NMDA receptors. Foods highest in glycine include gelatin, bone broth, poultry skin, and seafood. If you don’t get enough in your diet, you can get glycine as a powder and add it to a drink or yogurt (slightly sweet taste).
Related article: Glycine for slow wave sleep
Magnesium:
Magnesium is the off switch for NMDA receptors, preventing them from firing too much. Having sufficient magnesium is important for balanced glutamate. Check your diet using cronometer.com. I’ve often seen it written that everyone needs to take magnesium – the soil is depleted and we aren’t getting enough. However, studies show that about half of us don’t get enough magnesium, which means that half of us do get plenty of magnesium. So, you may not automatically need more.
Genetic variants can influence how much magnesium you need. Read the full article on magnesium here.
Gene | RS ID | Effect Allele | Your Genotype | Notes About Effect Allele |
---|---|---|---|---|
TRPM6 | rs3750425 | T | -- | lower serum magnesium levels |
TRPM6 | rs2274924 | C | -- | lower serum magnesium levels |
TRPM6 | rs121912625 | A | -- | rare mutation linked to hypomagnesia |
TRPM7 | rs8042919 | A | -- | increased sensitivity to low magnesium levels, |
CNNM2 | rs12413409 | A | -- | decreased risk of hypertension and heart disease (good!) |
CNNM2 | rs11191548 | C | -- | decreased risk of hypertension (good!) |
CNNM2 | rs7914558 | G | -- | GG only: decreased gray matter (on average) |
ATP2B1 | rs7965584 | G | -- | lower serum magnesium levels |
SHROOM3 | rs13146355 | A | -- | slightly higher serum magnesium levels |
SLC41A1 | rs708727 | A | -- | increased risk of Parkinson’s (possibly indicates less magnesium in the brain) |
SLC41A1 | rs823156 | G | -- | protective against Parkinson’s (possibly indicates more magnesium in the brain) |
Electrolytes:
Sodium, potassium, and chloride are all involved in glutamate transport out of the synapse and into the glial cells (EAAT2, EAAT1). Sodium and potassium are also involved in neuronal transmission with the ionotropic glutamate receptors (NMDA, AMPA). Using cronometer.com you can track to see what your daily intake of sodium, chloride, and potassium is. If you are regularly avoiding salt, you could experiment to see what happens to your brain with a little more salt. Keep track – see how more or less salt affects your brain function (memory, sleep, cognitive function). Similarly, increasing potassium may be helpful. Coconut water is a good source of potassium.
Lifestyle and Diet:
Foods that contain glutamate:
Foods that naturally contain glutamate include cheese (parmesan and Roquefort are the highest), walnuts, processed meats, tomato sauce, anchovies, mushrooms, fish sauce, soy sauce, and peas. MSG is monosodium glutamate, which increases glutamate levels in the brain. [ref]
Does dietary glutamate affect glutamate in the central nervous system? This isn’t entirely clear. Some studies show that it has no real effect.[ref] However, people who are prone to migraines can be sensitive to foods containing high levels of glutamate.[ref]
Stress increases glutamate in negative ways:
Chronic stress increases glutamate release to the point of possibly causing neuroinflammation in the hippocampus region of the brain. However, chronic stress also reduces glutamate in areas of the brain related to attention and memory.[ref]
Exercise increases both glutamate and GABA:
Studies show that physical exercise increases both glutamate and GABA levels, which is why it can cause you to feel both energized and calm. Higher exercise levels over the prior week correlate to higher resting glutamate levels.[ref]
Nutrients that affect glutamate:
Your diet also influences glutamate activity in the brain:
- Arachidonic acid: Arachidonic acid is an omega-6 fatty acid that can have pro-inflammatory properties. Astrocytes and neurons can produce arachidonic acid when stimulated by glutamate. Animal studies show that higher arachidonic acid levels decrease EAAT1 function and enhance EAAT2. [ref][ref]
- DHA: DHA is an omega-3 fatty acid that we get from fish (or marine oils). Animal studies show that DHA increases EAAT2 and EAAT3 levels and decreases EAAT1.[ref]
- Zinc: Zinc ions (Zn2+ ) affect both glutamate transporters and receptors (e.g. NMDA, kainate receptors).[ref]
Recap of your genes:
Gene | RS ID | Effect Allele | Your Genotype | Notes About Effect Allele |
---|---|---|---|---|
SLC1A1 | rs2228622 | A | -- | increased relative risk of OCD |
SLC1A1 | rs301430 | C | -- | higher expression, more anxiety in autism spectrum disorder; increased OCD risk |
SLC1A2 | rs3794087 | T | -- | increased relative risk of essential tremor |
SLC1A2 | rs12294045 | T | -- | increased relative risk of schizophrenia |
SLC1A2 | rs4354668 | G | -- | G/G: lower EAAT2 expression, impaired prefrontal cognitive performance in schizophrenia patients |
SLC1A2 | rs10768122 | G | -- | increased relative risk of vitiligo |
SLC1A2 | rs1570216 | C | -- | lower EAAT2 and higher glutamate levels in the anterior cingulate cortex, more rapid cycling in depressed patients. |
GRIA1 | rs548294 | T | -- | increased relative risk of migraines without aura |
GRIA3 | rs3761555 | C | -- | increased relative risk of migraines with aura |
GRIA3 | rs687577 | A | -- | longer sleep duration, on average |
GRIN2B | rs1806201 | A | -- | more likely to use or abuse ketamine; less flexible in decision-making; increased risk of Alzheimer’s disease |
GRIN2B | rs7301328 | C | -- | associated with disruptive behavior; increased risk of impulse control disorder (ICD) |
GRIN2B | rs1805502 | G | -- | increased relative risk of treatment-resistant depression |
GRIN2B | rs1805476 | T | -- | increased risk of cleaning subtype of OCD |
GRIK1 | rs2832407 | A | -- | altered kainate receptor function, little response to topiramate for reducing drinking |
GRIK2 | rs4840200 | C | -- | decreased risk of epilepsy |
GRIK2 | rs2227283 | A | -- | increased relative risk of aggressive behavior in bipolar disorder; increased relative risk of migraines |
GRIK2 | rs3213607 | A | -- | increased relative risk of epilepsy; lower risk of autism |
GRID2 | rs1030757 | C | -- | increased relative risk of OCD |
GRID2 | rs5019028 | G | -- | increased relative risk of OCD |
GRM5 | rs10831496 | A | -- | increased relative risk of severe Covid |
GRM6 | rs2067011 | A | -- | increased relative risk of autism spectrum disorder |
SLC3A2 | rs4726 | T | -- | less physical decline in the elderly (faster walking) |
GRIN2B | rs10193895 | C | -- | lower GRIN2B activity, increased relative risk of schizophrenia |
Related articles and topics:
Glutamate: Synthesis, transport, and supplement interactions
Dopamine Receptor SNPs: Addiction, Mood, ADHD, and Schizophrenia